Lesson 3:
The Essence of Life
|
3.3
What Living Things Have In Common
|
 |
Figure 3.3.1
Artist's conception of the first seas
of early Earth where life may have began.
|
If we are to reconstruct the Origin of Life we are first bound to admit
that there was a privileged period on Earth when life forms first
appeared, and that later forms then evolved from these earlier ones. If
this is so, several questions arise. Was there just one life form at the
beginning among many possible other ones? Did the form or forms that
became the ancestor or ancestors to all succeeding life forms emerge by
competition or by luck? As a single survivor? Or as an alliance between
several different early life forms? How do we get from self-replicating
molecules to self-replicating cells? Is this such a rare event that it
might only have happened once, or are there other places in the universe
where it did or could have happened? Are we, that is Life on Earth,
extremely special?
There are no fossils from the earliest history of Earth that will give
us clues to these questions. However, we might pick up such clues from
the things living organisms have in common. A common ancestor should
have had those very attributes.
First, there is the trivial fact, already mentioned, that organisms have
pretty much the same elementary composition. The chemistry of Life is
carbon chemistry, in combination with hydrogen, oxygen and nitrogen, and
some sulfur and phosphorus. As a rule, these elements are quite readily
available on Earth. Everyone uses them, and everyone uses them in pretty
much the same way. Given that we have the same general chemical
machinery, the pathogenic microbes we call germs can try to take ours
over for their purposes. In response, we (our defensive cells) eat them.
We know their chemistry (in our genes) and we know how to deal with it.
Second, all living organisms exist as cells or agglomeration of cells. A
cell has a boundary between itself and the outside world. In
single-celled organisms (which make up the great bulk of organisms on
our planet) the cell walls are relatively thick and robust. With a few
notable exceptions, single cells are too small to see with the unaided
eye. We call them "microbes" for that reason. Most of them are various
types of bacteria. (The biggest bacteria in fact can be seen by the
naked eye; they occur in black marine muds in anaerobic environments and
make a several mm long sheath, a housing wherein they live.) Other
single-celled organisms are "eucaryotic" cells, with their genetic
material collected in a true nucleus ("eu" means good, "caryos" means
core; both Greek). Typical examples are abundant in puddles, rivers,
lakes, and in the ocean, among the microscopic "plankton" organisms
suspended in the water. Among these are all sorts of cells moving by
means of rotating whips and hair-like protuberances.
We don't know how the first cell wall was made, but we can readily
appreciate the usefulness of the invention. Once the genetic
instructions were enclosed in a protected environment, it was like having
a patent on whatever successful adaptations were developed. Favorable
mutations that gave the individual an increased chance for survival
would pay off in a big way. This information would be passed on to
its offspring genetically.
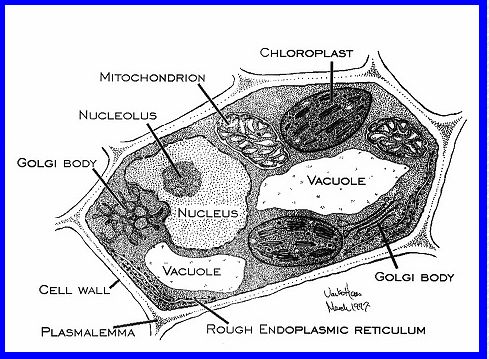 |
 |
Figure 3.3.2
A section through a eucaryotic cell, with its prominent nucleus, in
which the long DNA molecules (carrier of genetic instructions) lie
tangled up. Other prominent cell components or organelles are floating
in the endoplasmic fluid between the nucleus and the outer cell wall.
These organelles include the mitochondria (responsible for energy
budget), lysosomes (digestion), the reticulum and the Golgi apparatus.
The human body is made of eucaryotic cells, about 100,000,000,000 of
them. (Yes, 100 billion. As many as there are stars in the galaxy. The
human body also hosts bacteria, mainly in the guts. That number is even
larger.) |
Walled-in organisms could readily face new challenges, through
adaptation to a changing environment. Some, indeed many, of these
environmental changes would have been produced by the activities of the
living organisms themselves. Thus, Life had to play catch-up with the
very changes it wrought. (We call this the "Gaia-Playing-Catch-Up"
hypothesis, or "Catch-up" hypothesis for short.) The main example for
"catch-up" is the increasing availability for oxygen during the Archean
and Proterozoic, which led to the evolution of oxygen-using microbes,
and ultimately to the ascent of the energy-hungry eucaryotes.
If there ever was an alternative chemistry, or an alternative to cell
structure, or an alternative to passing on information to the next
generation by transferring RNA and DNA, we don't know about it. The
winners write the history books.
|